Microbial Fuel Cells: Generating Electricity from Waste
According to the Environment Impact Assessment (EIA), the global energy demand is projected to grow 47% in the next 30 years, owing to the rising population and rapid economic growth in developing countries. Rising global energy consumption, depleting origins of fossil fuels, and growing concerns about carbon emissions are creating a grave need for finding eco-friendly, alternate, and renewable energy sources. Microbial fuel cells (MFCs) have been gaining a lot of traction as they hold an immense potential to address three major sustainability issues confronting the world today—energy security, global warming, and wastewater management. Microbial fuel cells are bio-electrochemical devices that have the ability to produce electricity by converting biodegradable organic matter to electrical energy leveraging exoelectrogenic bacteria as catalyst. Exoelectrogenic bacteria are microorganisms that can extract electrons from the energy source and transfer them to the anode through extracellular electron transfer mechanisms and thus generate electrical current in microbial fuel cells.
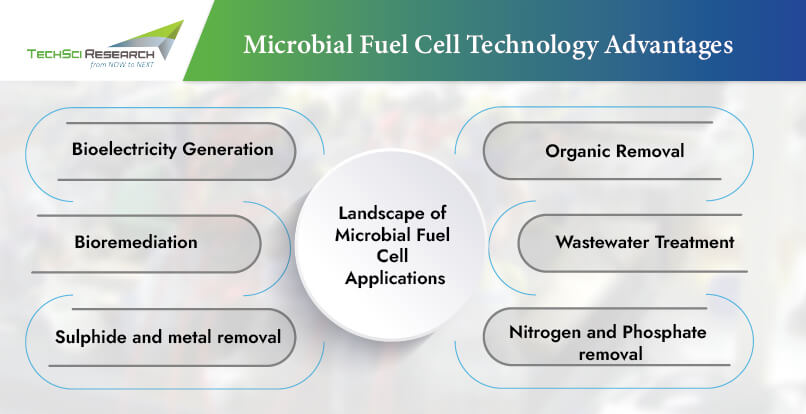
Composition & Working of a Typical MFC
A typical microbial fuel cell consists of several components that are divided into two chambers, anode and cathode, by a proton exchange membrane (PEM). Anaerobically degraded substrate is provided in the anodic chamber to release electrons, protons, and carbon dioxide. The electrons are transferred from anode to cathode employing external electrical circuit to generate electricity. At the cathode, protons and electrons are consumed, combined with oxygen to form into water.
Sustainable Wastewater Treatment and Contaminant Removal through Microbial Fuel Cell
Microbial fuel cells have emerged as a promising technology for pollutant removal and sustainable wastewater treatment. The working of MFC is based on electron donation and acceptance. Contaminants can be eliminated either as electron acceptors through reduction at the cathode or as electron donors through microbially catalysed oxidation at the anode. Besides, some contaminants can also function as electron mediators at the anode or cathode. The biodegradation of organic matter contained in wastewater are easily degraded under anaerobic conditions at the anode. The exoelectrogenic bacteria use their electron transport capacity to generate electricity from chemical energy.
The MFC technology outperforms the aerobic biological treatment based on activated sludge processes in eliminating intensive aeration and minimizing a large amount of generated sludge. Additionally, one of the biggest limitations associated with conventional wastewater removal is removing nitrogen, critical component in wastewater. However, MFCs can integrate nitrogen removal process into the process through biochemical reactions and convert organic nitrogen into ammonium through the ammonification process by heterotrophic bacteria. Some of the ammonium is utilized as nitrogen source for synthesizing biomass and the rest is removed through nitrification and denitrification process.
Generally, microbial fuel cells employ two types of microorganisms for electrode reducing and oxidizing, The electrode reducing microorganisms carry out reduction of electrode and facilitate movement towards anode whereas microbes involved in the oxidation, also known as electricigens, allow electron transfer. Electron oxidizing microorganisms receive various substances such as acetate and carbon dioxide. Microorganisms used for electricity generation through MFC are either pure cultured such as Geobacter and Shewanella species or mixed cultures.
Types of Substrates Used in MFCs
Substrates that can be used in MFCs for electricity production are as follows.
Human Waste
In future, toilets can be potential power stations as the biodegradable matter found in urine and faeces can be converted into electricity. Human waste and urine contain significant levels of carbon and nutritional molecules. Urine contains 2% urea by weight and human faeces have significant carbonaceous chemicals that have the potential to release electrons during microbial oxidation. In Ghana, MFC Latrine executed was able to generate 268 nW/m2 of electricity. The electricity was able to power an LED light inside the latrine while removing nitrogen from urine and compositing the faeces.
Plants
Plant exudes waste products through their roots into the soil layer known as rhizosphere. Bacteria chow down on plants’ sloughed-off cells along with proteins and sugars. As long as the plant lives, the bacteria can continue to feed on proteins and sugars, and the fuel cell can generate power. In plant MFCs, the protons are transferred through a membrane and recombine with oxygen to facilitate electron transfer in the circuit. Connecting a load in the circuit, electricity can be generated.
A few start-up companies such as Robial and Plant-e are working towards making microbial fuel cells, which can even be used to generate electricity in regenerative life support systems during long-term human space missions. Researchers are investigating ways to scale up reactors for increasing the electricity output. In 2021, Toyota Corporation launched a new hydrogen production facility for the launch of its second-generation microbial fuel-cell car.
Challenges Associated with the Commercialization of MFCs
One of the most difficult challenges associated with the commercialization of MFCs is setting up of efficient and cost-effective design for scaled-up versions of laboratory size reactors. Many aspects need to be addressed to duplicate the results of decontamination and electricity production such as separator’s configuration, mechanical strength, electrode cost, manner of oxidant supply, energy and space requirements, and so on. Most microbial electrochemical processes are based on oxidation-reaction reactions, which are affected by many operational variables. Low power output for direct application is a major problem for MFCs. When it comes to removing contaminants, MFCs have a quick execution time. Hence, the key to deploying MFCs for actual applications is to improve long-term operational stability. More research is required to better understand the operational mechanism of MFC system to improve power generation while enhancing financial stability. Currently, microbial fuel cells are able to generate electricity to power small devices such as small fans, LEDs, calculators, etc. But, if the technology is scaled, it holds a great promise to change the future of energy generation. Moreover, high cost of the components related to microbial fuel cells and low efficiency are expected to hinder the commercialization of MFCs.
Way Ahead
The world population is projected to reach 9.5 billion by 2050. Given that most of our energy needs are still being fulfilled by fossil fuels, this creates significant challenges when it comes to generating and providing sustainable electricity while combating climate change. Environmental regulatory authorities are pushing researchers to create and offer novel MFC techniques and technologies for the betterment of human and environment. According to Techsci Research report on “Automated Waste Collection System Market – Global Industry Size, Share, Trends, Opportunity and Forecast, 2017-2027, Segmented By Type (Gravity System and Full Vacuum System), By Operation (Stationary, and Mobile), By Application (Airports, Healthcare Facilities, Educational Institutions, Corporate Offices, Hotels/ Restaurants, Industries and Others) and By Region”, the global automated waste collection system market is anticipated to register significant growth in the coming years. The factors attributing to the growth are rapid urbanization in developing economies and growing adoption of automated waste collection systems in urban cities. Moreover, rising awareness pertaining to the benefits of cleanliness and increasing demand for cleaner and greener environment are projected to boost the growth of global automated waste collection system market in the coming years.